Mastering Material Selection in Product Design: Understanding Steel Variants and Effective Communication Strategies
- Aniekpeno Ifeh
- May 24, 2024
- 19 min read
Introduction
The selection of materials in product design is a decision fraught with complexity and nuance. The challenge is not just in choosing the right material for the job but also in understanding the intricate language of materials—their benefits, drawbacks, and specific characteristics. Take steel, for example; the differences between SGCC, SPCC, SUS 201, and SUS 401 may seem minor on paper, but they can have significant implications in practice.
This blog post discusses the world of material selection and the crucial role of clear communication in navigating this complex landscape.
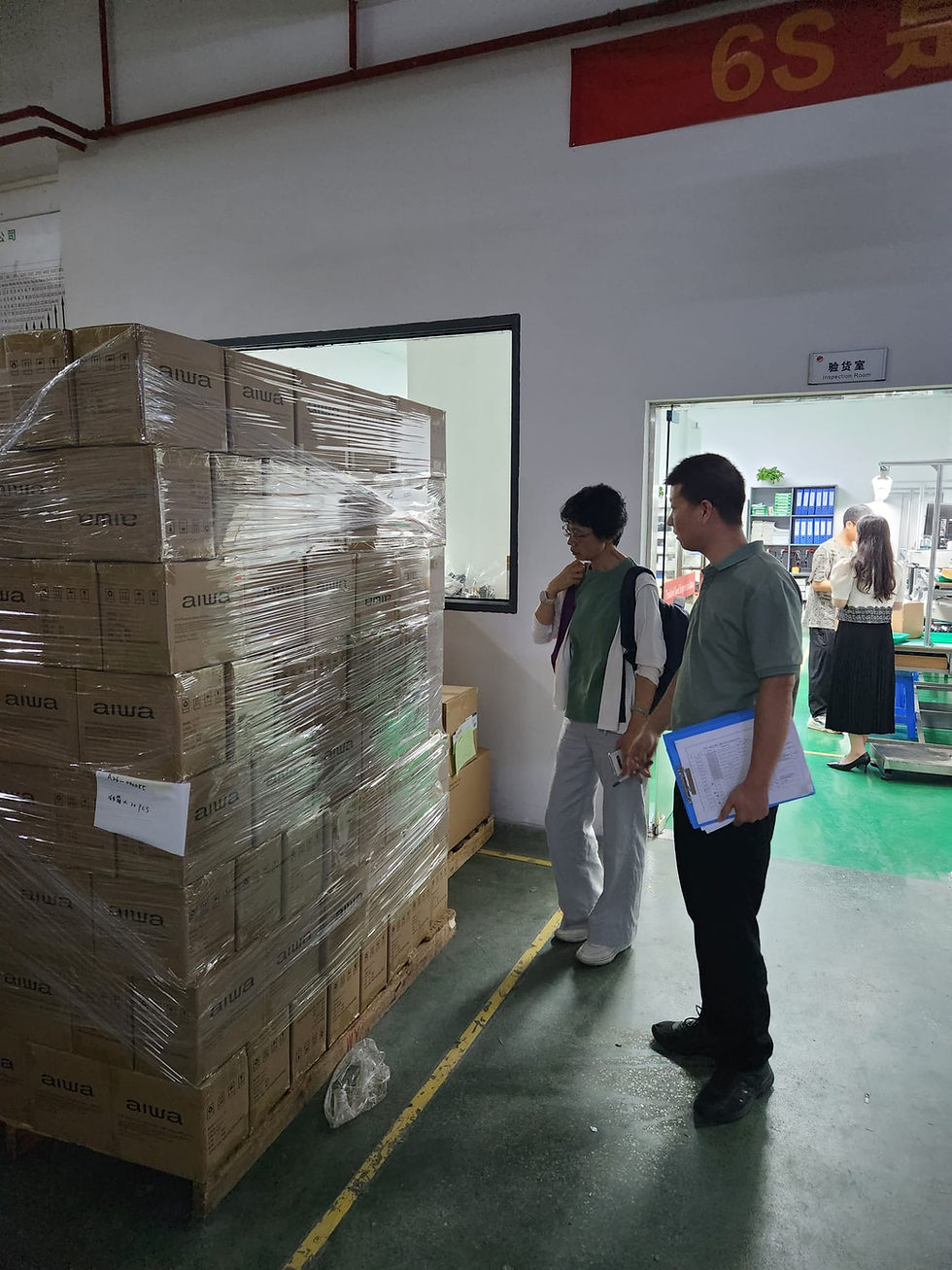
The Language of Material Selection
Material selection is a critical phase in product design, where decisions are made that affect the product's functionality, durability, cost, and even aesthetic appeal. However, the technical language used to describe materials can be confusing, especially when dealing with various alloys and grades, each with its unique properties and applications.
Technical Jargon
Technical jargon refers to the specialized language and terms used within a specific field or industry. In the context of materials science, this jargon can pose significant challenges in communication for several reasons:
Complexity Within Designs and Precision
Precision Required: Material specifications often require precise terminology to avoid misunderstandings. Terms like "ductility," "tensile strength," "yield point," and "modulus of elasticity" have very specific meanings that are critical to understanding a material's properties and performance.
Complex Concepts: Many concepts in materials science are inherently complex and cannot be easily simplified without losing essential information. For example, explaining the difference between "crystalline" and "amorphous" structures involves detailed knowledge of atomic arrangements and bonding.
Barriers to Understanding
Audience Knowledge: Stakeholders, such as product managers, designers, or clients, may not have a background in materials science. This disparity in knowledge can lead to misinterpretations if technical jargon is not adequately explained.
Learning Curve: Even for those within the industry, staying updated with evolving terminology and new material innovations can be challenging. Terms like "nanocomposites" or "bio-based polymers" might be relatively new and not universally understood.
Examples of Technical Jargon in Materials Science
Mechanical Properties: Terms such as "fatigue limit," "creep resistance," and "hardness" describe specific mechanical behaviours that require detailed understanding to interpret correctly.
Chemical Properties: Descriptions involving "corrosion resistance," "chemical stability," and "thermal conductivity" often rely on precise definitions that might not be intuitive for all stakeholders.
Material Grades and Standards: Various systems of grading and standards (e.g., ASTM, ISO) use highly specific terminology to define material properties, which can be difficult to navigate without specialized knowledge.
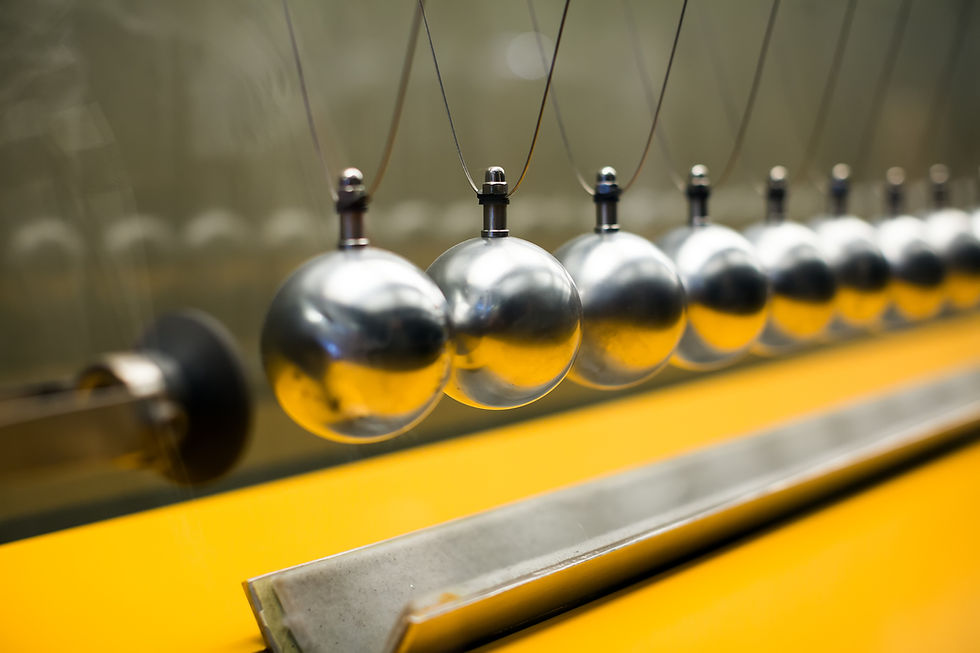
Overcoming Challenges
Simplification and Education: Simplifying complex terms and providing clear, accessible explanations can help bridge the gap. Using analogies and visual aids can make technical concepts more relatable and easier to understand.
Clear Documentation: Comprehensive and clear documentation, including glossaries of terms, can assist stakeholders in understanding material specifications. This documentation should be readily available and easy to navigate.
Effective Communication: Regular training sessions, workshops, and collaborative meetings can help ensure all team members are on the same page. Encouraging questions and fostering an environment where stakeholders feel comfortable seeking clarification can also mitigate misunderstandings.
Translation Tools: Utilizing translation tools and services can help convey technical terms in different languages accurately, ensuring international teams understand the specifications despite language barriers.
By addressing these challenges, teams can improve their communication regarding material specifications, leading to better collaboration, fewer misunderstandings, and more successful project outcomes.
Cultural and Language Differences
In the context of international collaborations and teams, cultural and language differences add another layer of complexity to the already challenging task of communicating material specifications. These differences can lead to misunderstandings, errors, and inefficiencies if not properly managed.
Differing Terminologies
Regional Standards: Different countries and regions often have their own standards and grading systems for materials. For example, steel grades in the United States are governed by ASTM standards, while European standards are defined by EN (European Norms) specifications. This can lead to confusion if team members are not familiar with the standards used by their counterparts.
Translation of Terms: Technical terms may not have direct translations in other languages, or the translations may not convey the precise meaning intended. For instance, the term "yield strength" in English might be translated differently in German or Japanese, leading to potential misinterpretations of material properties.
Measurement Systems
Metric vs. Imperial Units: Different countries use different measurement systems. The United States primarily uses the Imperial system (inches, pounds, Fahrenheit), while most other countries use the Metric system (meters, kilograms, Celsius). This discrepancy can cause confusion and errors in specifications if conversions are not handled accurately.
Local Practices: In addition to formal measurement systems, local practices and preferences can vary. For example, a material commonly used in one country might be less preferred or even unavailable in another, affecting design choices and availability.
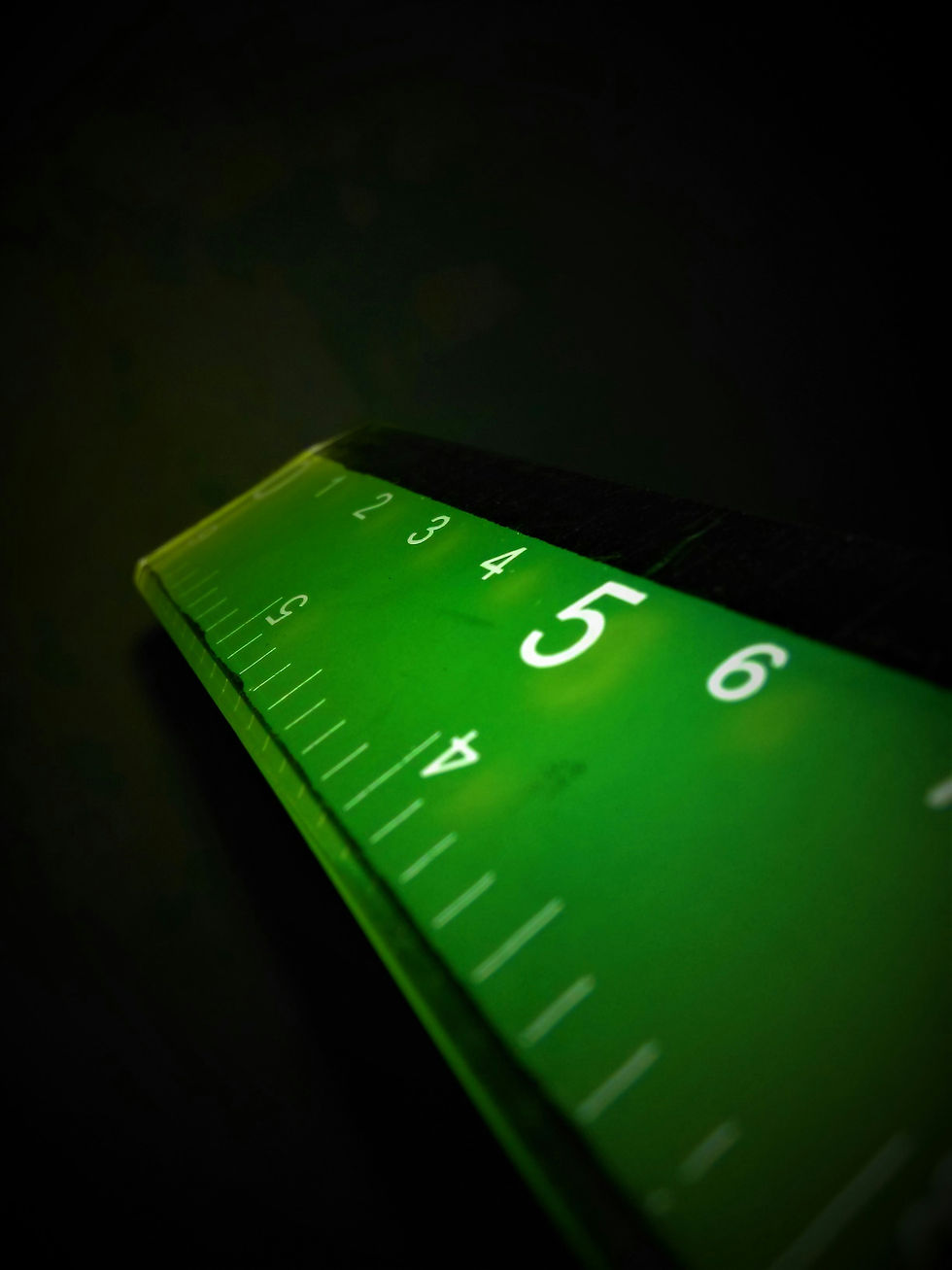
Language Barriers
Proficiency Levels: Team members from different countries may have varying levels of proficiency in the common language used for communication, typically English. This can lead to misunderstandings if complex technical jargon is used without adequate explanation.
Nuances and Idioms: Even when technical terms are translated accurately, nuances and idiomatic expressions might not carry over well. For example, a phrase like "tough as nails" might be confusing to non-native speakers if used to describe a material property informally.
Cultural Differences
Communication Styles: Different cultures have different communication styles. Some cultures may favour direct and explicit communication, while others may rely on context and implicit understanding. This can affect how material specifications are discussed and agreed upon.
Approach to Hierarchy and Decision-Making: Cultural attitudes toward hierarchy and decision-making can impact how information is shared and decisions are made. In some cultures, decisions might be made collectively with input from all team members, while in others, decisions might be top-down, affecting how material specifications are communicated and implemented.
Overcoming Challenges
Standardization and Documentation: Developing standardized documentation that includes both regional standards and conversions can help bridge gaps. Providing dual units (both Metric and Imperial) in specifications can reduce errors and misunderstandings.
Clear Translation and Glossaries: Using professional translation services and developing glossaries of technical terms in multiple languages can ensure that everyone is on the same page. These glossaries should be readily accessible to all team members.
Cultural Training and Awareness: Providing cultural training and encouraging awareness of different communication styles and practices can help teams navigate these differences more effectively. Understanding and respecting cultural norms can lead to more effective collaboration.
Regular Communication and Feedback: Encouraging regular and open communication, along with feedback loops, can help identify and resolve misunderstandings quickly. Tools like video conferencing, collaborative platforms, and instant messaging can facilitate ongoing dialogue.
Language Support: Offering language support, such as translation tools or language learning resources, can improve proficiency and confidence in communicating technical information.
By addressing cultural and language differences proactively, teams can enhance their communication regarding material specifications, leading to more effective collaboration and successful project outcomes.
Conveying Material Properties
Communicating the properties of materials effectively is critical in ensuring that all stakeholders understand the benefits, limitations, and appropriate applications of different materials. This challenge becomes more pronounced when dealing with non-technical audiences or interdisciplinary teams. Here are the key aspects and strategies for conveying material properties effectively:
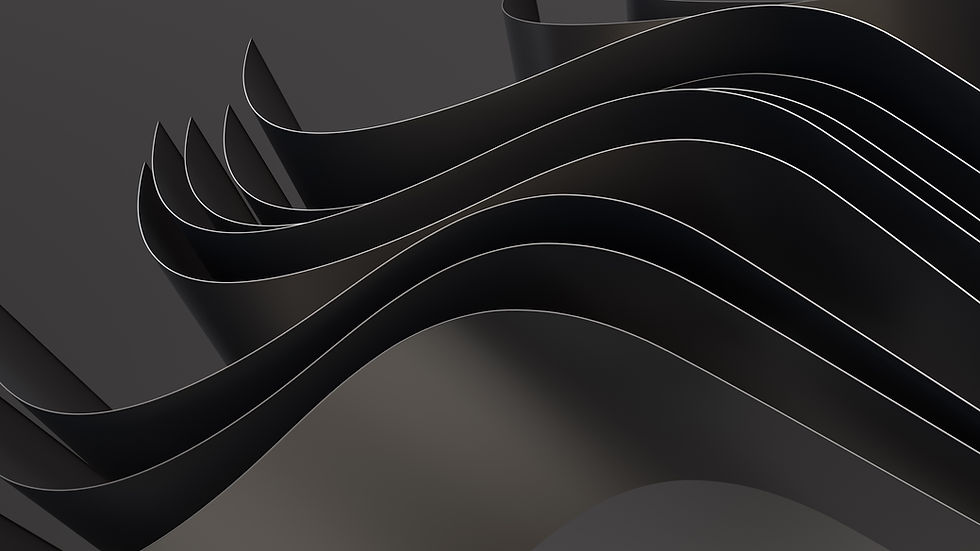
Understanding the Audience
Stakeholder Knowledge Levels: Different stakeholders have varying levels of technical knowledge. Engineers and materials scientists may understand detailed specifications and technical terms, while designers, project managers, or clients may not. Tailoring the communication to the audience’s knowledge level is essential.
Interdisciplinary Teams: In many projects, teams consist of members from various disciplines (e.g., engineering, marketing, finance). Each discipline has its focus and priorities, which can influence how they perceive material properties.
Clear and Accessible Communication
Simplification Without Loss of Meaning: Simplifying complex technical information without losing essential details is a delicate balance. Using plain language and avoiding unnecessary jargon can make the information more accessible.
Analogies and Comparisons: Using analogies and comparisons can help convey complex material properties. For instance, explaining the strength of a material by comparing it to a well-known material or using everyday examples can make the information more relatable.
Visual Aids: Charts, graphs, and diagrams can be extremely effective in conveying material properties. Visual representations can illustrate differences in properties like strength, flexibility, or thermal conductivity more clearly than text alone.
Key Material Properties to Convey
Mechanical Properties:
Strength: Describing tensile, compressive, and shear strength, often through comparison to familiar materials.
Elasticity and Plasticity: Explaining how materials deform under stress and return to their original shape.
Hardness: Communicating resistance to deformation and wear, possibly using the Mohs scale or other relatable measures.
Fatigue and Creep: Explaining how materials behave under cyclic loading and prolonged stress.
Thermal Properties:
Conductivity: How well a material conducts heat, important for thermal management in applications.
Expansion: Explaining how materials expand with temperature changes, is crucial for design considerations.
Electrical Properties:
Conductivity and Resistivity: Describing how materials conduct electricity, is important for electrical and electronic applications.
Dielectric Strength: Explaining a material's ability to withstand electrical breakdown.
Chemical Properties:
Corrosion Resistance: Describing a material’s ability to resist degradation from chemical reactions.
Chemical Stability: Explaining how a material maintains its properties in various chemical environments.
Strategies for Effective Communication
Layered Information: Providing information in layers, starting with a high-level overview and then delving into more technical details as needed, can help cater to different levels of understanding.
Interactive Tools: Utilizing interactive tools, such as simulations and material selection software, can allow stakeholders to explore material properties in a hands-on manner, improving comprehension.
Case Studies and Examples: Using real-world examples and case studies can illustrate how specific material properties impact performance in actual applications, making the information more tangible.
Feedback Mechanisms: Encouraging questions and feedback ensures that any misunderstandings can be quickly addressed. Regularly soliciting input from stakeholders can help refine the communication process.
Educational Resources: Providing supplementary educational resources, such as glossaries, explainer videos, and detailed documentation, can help stakeholders build their understanding over time.
Consistent Terminology: Ensuring that the terminology used is consistent throughout all communications and documentation can reduce confusion. If different terms are used, providing clear definitions and explanations is essential.
By employing these strategies, the communication of material properties can be significantly improved, leading to better-informed decisions and more effective collaboration among all stakeholders involved in a project.
Strategies for Effective Communication in Material Selection
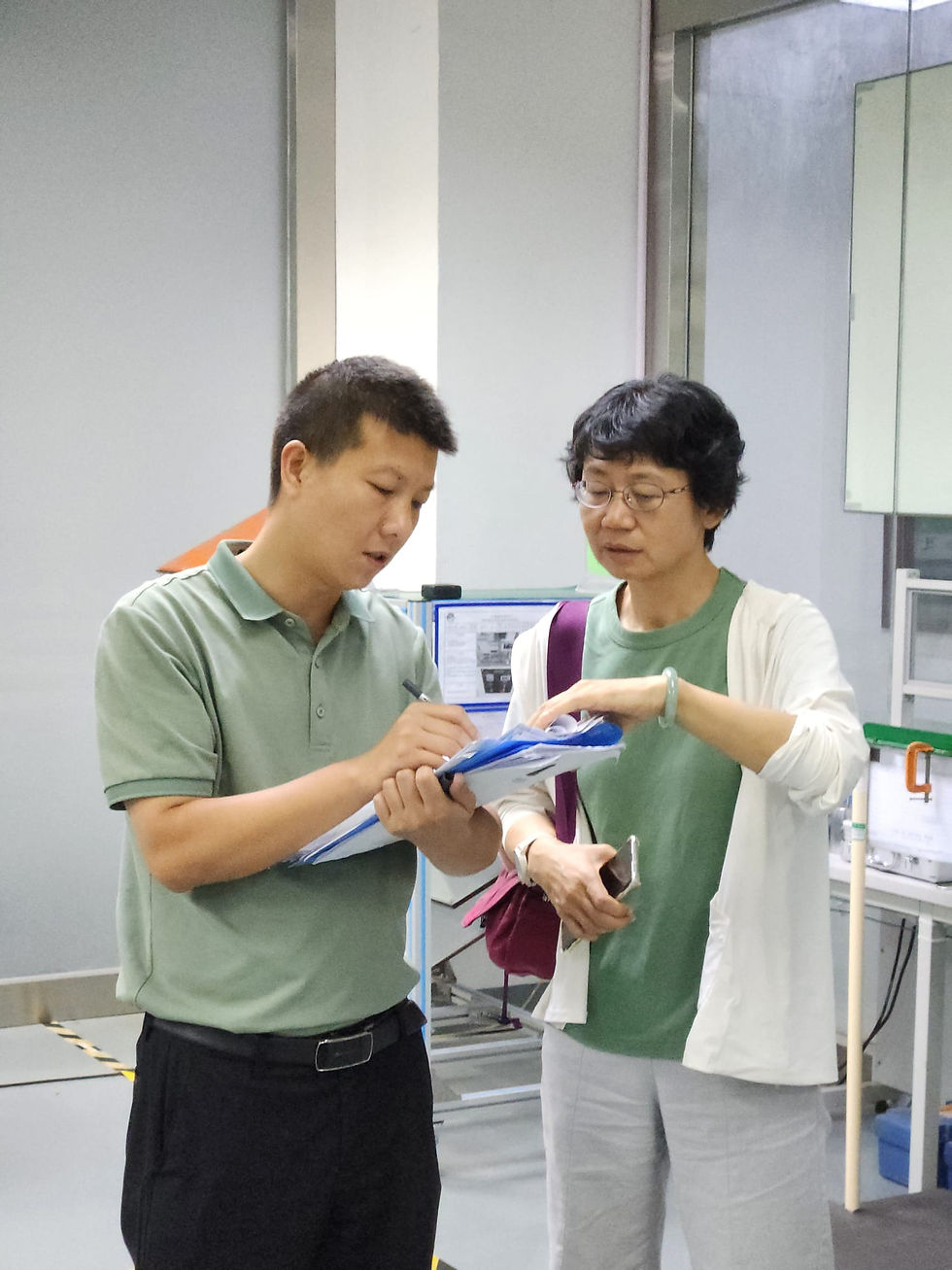
Use of Visual Aids
Visual aids are crucial tools in the material selection process, as they transform complex data and technical jargon into more understandable and accessible formats. Here are some specific types of visual aids and how they can be effectively used:
Diagrams
Phase Diagrams: These show the stability of different phases of a material at various temperatures and compositions. For example, an iron-carbon phase diagram helps in understanding the different phases of steel at different carbon contents and temperatures.
Microstructure Diagrams: Visual representations of the microstructure of materials (e.g., grain size, phase distribution) help in understanding how these structures impact properties like strength and ductility.
Charts and Graphs
Property Comparison Charts: These can compare properties such as tensile strength, hardness, corrosion resistance, and thermal conductivity across different materials. Spider charts (radar charts) are particularly effective in comparing multiple properties simultaneously.
Cost vs. Performance Graphs: These illustrate the trade-offs between the cost of materials and their performance characteristics, helping stakeholders balance budget constraints with performance requirements.
Stress-Strain Curves: These graphs show how a material deforms under stress, providing insights into its elasticity, yield strength, and ultimate tensile strength.
Comparative Tables
Material Property Tables: Tables listing properties of various materials in a side-by-side format make it easy to compare key attributes like density, modulus of elasticity, thermal expansion, and electrical conductivity.
Application Suitability Tables: These tables indicate the suitability of different materials for specific applications, considering factors like environmental conditions, load requirements, and regulatory standards.
3D Models and Simulations
Finite Element Analysis (FEA) Models: These provide a visual representation of how materials behave under different loading conditions, helping to predict potential failure points and optimize material selection for structural components.
Virtual Prototypes: 3D models that can be rotated, zoomed, and manipulated to show how different materials impact the overall design and functionality of a product.
Benefits of Using Visual Aids
Improved Understanding: Visual aids make complex material properties and interactions easier to grasp, especially for non-technical stakeholders.
Enhanced Communication: They facilitate clearer communication among team members, including engineers, designers, managers, and clients, ensuring everyone is on the same page.
Quicker Decision-Making: By providing a clear visual comparison, stakeholders can make more informed decisions more quickly, reducing the time spent in deliberation and discussion.
Greater Engagement: Visual elements tend to capture attention and interest more effectively than textual descriptions, leading to more engaged and participative discussions.
Implementation Tips
Simplify Visuals: Ensure that the visuals are not overly complex and are tailored to the audience's level of understanding.
Use Interactive Tools: Where possible, use interactive digital tools that allow stakeholders to explore different scenarios and outcomes dynamically.
Regular Updates: Keep the visual aids updated with the latest data and findings to ensure they remain relevant and accurate.
Combine with Narratives: Pair visual aids with clear, concise explanations to provide context and enhance comprehension.
By integrating these visual aids into the material selection process, teams can navigate the complexities of material properties more effectively, leading to better-informed decisions and more successful product outcomes.
Training and Education
Training and education play a vital role in empowering team members to make informed decisions about material selection. Here are some strategies and methods to enhance the understanding of materials science and its application in product design:
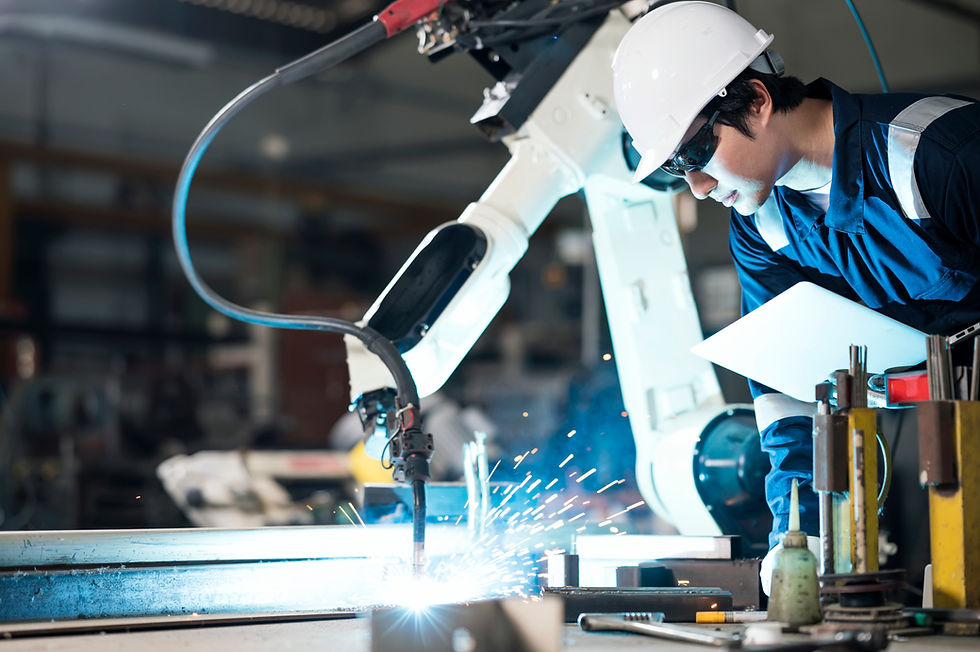
Foundational Courses
Materials Science Basics: Courses covering the fundamental concepts of materials science, including the properties of metals, polymers, ceramics, and composites, and their behaviours under different conditions.
Material Properties and Testing: Training on how to interpret material properties (e.g., tensile strength, hardness, thermal conductivity) and understanding various testing methods used to evaluate these properties.
Advanced and Specialized Training
Advanced Materials: In-depth courses on advanced materials such as nanomaterials, smart materials, and biomaterials, which are increasingly used in cutting-edge applications.
Industry-Specific Materials: Training focused on materials commonly used in specific industries (e.g., aerospace, automotive, electronics) to address particular challenges and requirements.
Workshops and Seminars
Interactive Workshops: Hands-on workshops where participants can engage in practical exercises, such as material testing, failure analysis, and selection exercises based on real-world scenarios.
Expert Seminars: Sessions led by industry experts who can share the latest developments, trends, and case studies in material science and engineering.
E-Learning and Online Resources
Online Courses and Webinars: Accessible online courses and webinars that allow team members to learn at their own pace, covering both foundational and advanced topics.
Virtual Labs: Online platforms that simulate laboratory experiments and material testing, providing a practical learning experience without the need for physical lab access.
Certifications and Continuous Learning
Certification Programs: Formal certification programs in materials science and engineering that validate the expertise and knowledge of team members.
Continuous Learning: Encouraging ongoing education through attending conferences, subscribing to industry journals, and participating in professional networks.
Benefits of Training and Education
Enhanced Competence: Team members gain a solid understanding of material properties and selection criteria, leading to more informed and confident decision-making.
Cross-Disciplinary Communication: Improved knowledge helps bridge the gap between different disciplines (e.g., engineering, design, procurement), fostering better collaboration.
Innovation and Creativity: With a deeper understanding of materials, team members can explore innovative uses and combinations of materials to enhance product performance and aesthetics.
Risk Reduction: Proper training helps in identifying potential material failures and selecting materials that mitigate risks, improving product reliability and safety.
Implementation Tips
Customized Training Programs: Tailor training programs to address the specific needs and knowledge levels of different team members, from novices to experienced professionals.
Blended Learning Approach: Combine in-person training with online resources to provide a flexible and comprehensive learning experience.
Regular Updates: Keep training content current with the latest advancements in materials science and industry practices.
Encourage Collaboration: Foster a culture of continuous learning and knowledge sharing among team members through regular meetings, discussion forums, and collaborative projects.
Feedback Mechanisms: Implement feedback systems to continuously improve training programs based on participant input and evolving industry requirements.
Investing in training and education ensures that team members are well-equipped to navigate the complexities of material selection. This investment not only enhances individual capabilities but also strengthens the overall effectiveness and innovation potential of the product development process.
Collaborative Decision-Making Tools
Collaborative decision-making tools facilitate teamwork and enhance communication among stakeholders involved in the material selection process. These tools enable teams to share insights, discuss options, and reach consensus more efficiently. Here’s a detailed look at different types of collaborative decision-making tools and their benefits:

Digital Platforms and Software
a. Material Selection Software:
Granta MI: A comprehensive materials information management system that helps in storing, analyzing, and comparing material data. It supports collaboration by allowing multiple users to access and contribute to the database.
CES Selector: This software helps in comparing materials based on various properties and performance criteria. Teams can use it to visualize trade-offs and make informed decisions.
b. Project Management Tools:
Trello: A visual project management tool that uses boards, lists, and cards to organize tasks. Teams can create boards dedicated to material selection projects, track progress, and assign responsibilities.
Asana: A collaborative work management platform where teams can create projects, set milestones, and monitor tasks related to material selection.
Collaborative Online Workspaces
a. Google Workspace:
Tools like Google Docs, Sheets, and Slides allow real-time collaboration on documents, spreadsheets, and presentations. Team members can comment, suggest edits, and track changes, ensuring everyone stays informed and aligned.
b. Microsoft Teams:
A hub for teamwork that integrates with Office 365. It includes features like chat, video meetings, file sharing, and collaborative document editing, making it easier for teams to work together on material selection.
Communication and Brainstorming Tools
a. Slack:
A messaging platform that supports organized communication through channels. Teams can create dedicated channels for material selection discussions, share files, and integrate with other tools for seamless collaboration.
b. Miro:
An online whiteboard tool that allows teams to brainstorm and collaborate visually. It supports mind mapping, flowcharts, and sticky notes, which can be used to map out material selection processes and decision criteria.
Data Visualization and Analysis Tools
a. Tableau:
A data visualization tool that helps in creating interactive and shareable dashboards. Teams can use it to visualize material properties, performance data, and cost analyses, facilitating data-driven decision-making.
b. Power BI:
A business analytics tool by Microsoft that provides interactive visualizations and business intelligence capabilities. It allows teams to create reports and dashboards to analyze material data collaboratively.
Decision Support Systems (DSS)
a. AHP (Analytic Hierarchy Process):
A structured technique for organizing and analyzing complex decisions, based on mathematics and psychology. Tools like Expert Choice implement AHP to help teams prioritize material selection criteria and evaluate options systematically.
b. Decision Matrix Tools:
Tools that use a decision matrix to evaluate and compare multiple materials against a set of predefined criteria. Excel and specialized software like Decision Matrix and Priority Matrix can assist in this process.
Benefits of Collaborative Decision-Making Tools
Enhanced Communication: These tools facilitate clear and continuous communication among team members, reducing misunderstandings and ensuring that everyone is on the same page.
Increased Engagement: By involving all relevant stakeholders in the decision-making process, these tools help in harnessing diverse perspectives and expertise, leading to more comprehensive and balanced decisions.
Efficiency and Productivity: Collaborative tools streamline workflows and automate many aspects of data management and analysis, saving time and effort.
Improved Transparency: The use of collaborative tools ensures that all decisions and rationale are documented and accessible, promoting transparency and accountability.
Data-Driven Decisions: By providing powerful data analysis and visualization capabilities, these tools enable teams to make informed, evidence-based decisions.
Implementation Tips
Choose the Right Tools: Select tools that fit the specific needs and workflow of your team. Ensure they are user-friendly and integrate well with existing systems.
Provide Training: Ensure all team members are adequately trained to use the selected tools effectively. Provide ongoing support and resources to address any issues.
Encourage Adoption: Foster a collaborative culture by encouraging the regular use of these tools in everyday workflows. Highlight their benefits to gain buy-in from all team members.
Set Clear Guidelines: Establish clear guidelines and best practices for using collaborative tools, including protocols for communication, data sharing, and decision-making processes.
Regular Review: Periodically review the effectiveness of the tools and make necessary adjustments based on team feedback and evolving needs.
Collaborative decision-making tools are essential for modern material selection processes, enabling teams to leverage collective expertise, streamline workflows, and make informed decisions. By integrating these tools into their workflows, organizations can enhance collaboration, improve decision quality, and ultimately drive better product outcomes.
Consultation with Material Experts
Consulting with material experts is a critical component of the material selection process. Experts bring specialized knowledge and experience that can significantly enhance the decision-making process, ensuring that the materials chosen meet all necessary criteria for performance, cost, and manufacturability. Here’s a detailed look at the benefits and strategies for effective consultation with material experts:
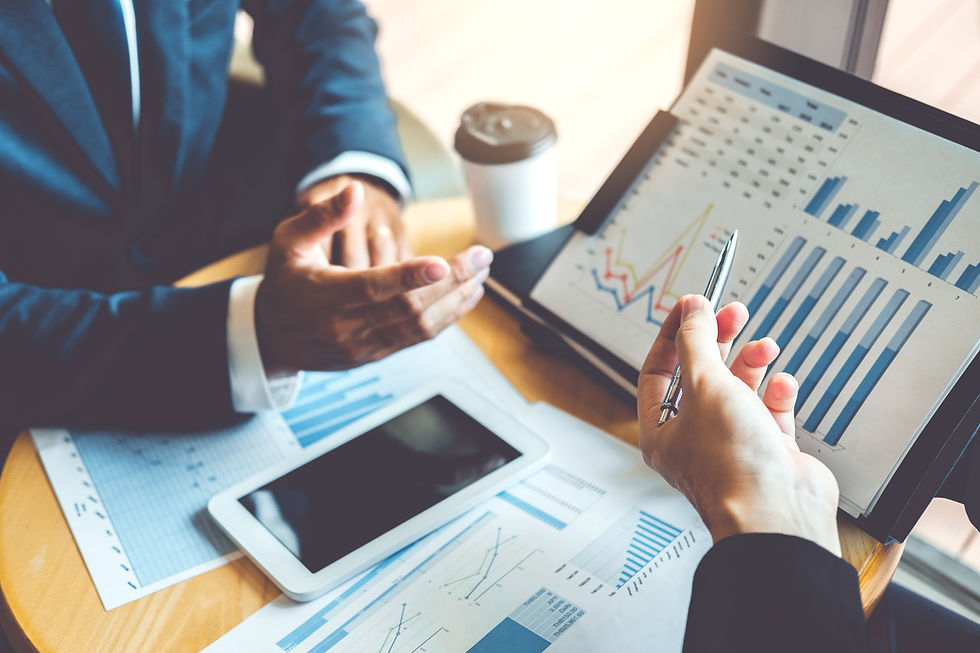
Roles of Material Experts
a. Materials Scientists and Engineers:
Materials Scientists: Experts in the fundamental properties and behaviours of materials, including metals, polymers, ceramics, and composites. They understand the microscopic and macroscopic properties that influence material performance.
Materials Engineers: Professionals who apply materials science principles to design and develop new materials and processes. They often work on practical applications and can provide insights into manufacturability and real-world performance.
b. Industry Specialists:
Experts with specific knowledge related to particular industries (e.g., aerospace, automotive, electronics) can provide insights into industry-specific standards, regulations, and best practices.
c. Academic Researchers:
Researchers from universities and research institutions often work on cutting-edge developments in materials science. Their insights can be invaluable for innovative projects requiring advanced materials.
Benefits of Consulting Material Experts
a. In-Depth Knowledge:
Experts possess a deep understanding of material properties, behaviours, and limitations, helping to avoid common pitfalls and select the best materials for specific applications.
b. Innovation and Problem-Solving:
Experts can introduce new materials or innovative solutions that may not be widely known, potentially improving product performance and opening up new possibilities.
c. Risk Mitigation:
By understanding potential failure modes and material weaknesses, experts can help design more robust products and select materials that minimize risks and enhance safety.
d. Cost Efficiency:
Experts can identify cost-effective materials and processes, optimizing the balance between material performance and budget constraints.
e. Regulatory Compliance:
Experts ensure that selected materials meet relevant industry standards and regulatory requirements, avoiding legal and compliance issues.
Strategies for Effective Consultation
a. Identify the Right Experts:
Determine the specific expertise needed based on the project’s requirements. This might include materials scientists, engineers, industry specialists, or academic researchers.
b. Collaborative Engagement:
Engage experts early in the design process and encourage ongoing collaboration. Regular meetings and open communication channels help integrate their insights effectively.
c. Clear Objectives:
Clearly define the goals and requirements of the project when consulting with experts. This includes performance criteria, environmental conditions, budget constraints, and any specific challenges or concerns.
d. Utilize Diverse Expertise:
Consult multiple experts with diverse backgrounds to gain a well-rounded perspective. This approach helps in identifying the best material solutions and avoids potential biases.
e. Documentation and Knowledge Sharing:
Document all consultations and recommendations from experts. Share this knowledge with the entire team to ensure everyone is informed and aligned.
f. Continuous Learning:
Encourage team members to learn from experts and stay updated on the latest developments in materials science. This can be facilitated through workshops, seminars, and ongoing training sessions.
Practical Approaches to Consultation
a. Advisory Boards:
Establish advisory boards consisting of material experts who can provide ongoing guidance and support for multiple projects.
b. Consultancy Services:
Hire specialized consultancy firms or independent experts on a project basis to address specific material selection challenges.
c. Partnerships with Academic Institutions:
Collaborate with universities and research institutions to leverage their expertise and access to cutting-edge research.
d. Industry Conferences and Workshops:
Attend conferences, seminars, and workshops to network with experts, gain insights into the latest trends, and discuss specific material challenges.
e. Online Expert Networks:
Utilize online platforms and networks (e.g., LinkedIn, ResearchGate) to connect with material experts and seek advice on specific issues.
Consulting with material experts is essential for making informed, strategic decisions in material selection. By leveraging their specialized knowledge and experience, teams can enhance product performance, ensure regulatory compliance, and optimize cost efficiency. Implementing effective consultation strategies, including early engagement, clear communication, and diverse expertise, helps in achieving the best outcomes for material selection projects.
Understanding Steel Variants: SGCC, SPCC, SUS 201, SUS 401
SGCC (Galvanized Steel): SGCC refers to galvanized steel, which is steel coated with a layer of zinc to prevent corrosion. This makes it ideal for outdoor applications or environments where corrosion resistance is essential.
SPCC (Cold Rolled Steel): SPCC stands for cold-rolled steel, known for its fine surface finish and higher strength. It's commonly used in automotive parts, electronic appliances, and precision instruments.
SUS 201 (Austenitic Stainless Steel): SUS 201 is a type of austenitic stainless steel that is lower in cost but higher in manganese, offering good formability and corrosion resistance. It's often used in cookware, hose clamps, and architectural applications.
SUS 401 (Martensitic Stainless Steel): SUS 401 is a martensitic stainless steel known for its hardness and high strength, making it suitable for applications requiring wear resistance like surgical instruments and cutlery.

SGCC (Galvanized Steel)
Strengths:
Corrosion Resistance: The zinc coating on SGCC steel provides excellent protection against rust and corrosion, making it ideal for outdoor or humid environments.
Paintability: SGCC can be easily painted, allowing for aesthetic versatility.
Workability: It can be cut, formed, and joined easily, making it suitable for a wide range of fabrication processes.
Weaknesses:
Limited Durability Under Heavy Stress: While corrosion-resistant, SGCC is not the strongest steel and may deform under heavy loads or impact.
Zinc Layer Wear: Over time, the zinc layer can wear off, especially in harsh conditions, reducing its corrosion resistance.
SPCC (Cold Rolled Steel)
Strengths:
Surface Finish: SPCC provides a smooth surface finish, ideal for applications where aesthetics are important, like consumer electronics.
Strength: Cold rolling increases the yield strength, tensile strength, and hardness of the steel, making SPCC stronger than hot-rolled steel.
Precision: SPCC allows for high precision in dimensions, essential for intricate or detailed work.
Weaknesses:
Cost: The process of cold rolling is more expensive compared to hot rolling, impacting the overall cost of the material.
Brittleness: Increased strength comes with reduced elasticity, making SPCC more prone to cracking under certain forming operations.
SUS 201 (Austenitic Stainless Steel)
Strengths:
Corrosion Resistance: Good resistance to water, rust, and a variety of chemicals.
Formability: Excellent formability makes SUS 201 easy to shape and manufacture into various products.
Cost-Effectiveness: Generally cheaper than other types of stainless steel, like SUS 304.
Weaknesses:
Lower Nickel Content: Less nickel compared to other austenitic steels makes it less resistant to corrosion and impact in harsh environments.
Magnetic Properties: Unlike other austenitic steels, SUS 201 can exhibit magnetic properties after cold working.
SUS 401 (Martensitic Stainless Steel)
Strengths:
High Hardness and Strength: SUS 401 is hardened through heat treatment, giving it high strength and wear resistance.
Magnetic: Can be used in applications where magnetic properties are desirable.
Moderate Corrosion Resistance: Offers decent resistance to corrosion, though not as high as austenitic or ferritic stainless steels.
Weaknesses:
Limited Weldability: Due to its hardening properties, SUS 401 is less weldable than other stainless steels.
Lower Corrosion Resistance than Austenitic Stainless Steels: While it does provide some corrosion resistance, it is not as effective as austenitic stainless steels in highly corrosive environments.
Each of these materials serves specific industrial needs based on their unique strengths and weaknesses. The selection of a particular type of steel should be guided by the specific requirements of the application, such as the need for corrosion resistance, strength, formability, or cost-effectiveness. Understanding these properties is essential for making informed decisions in product design and manufacturing.
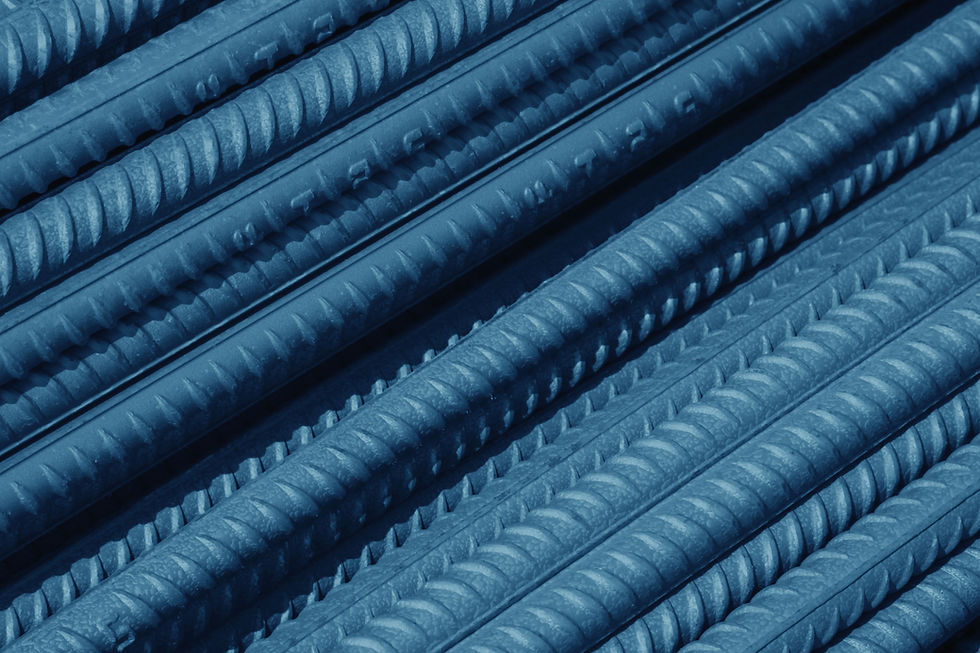
Conclusion
Navigating the intricate world of material selection in product design requires a deep understanding of both the materials themselves and the context in which they will be used. The nuanced differences between steel variants like SGCC, SPCC, SUS 201, and SUS 401 exemplify the importance of detailed knowledge and clear communication in making informed decisions.
Throughout this post, we've explored the critical aspects of material selection, emphasizing the challenges posed by technical jargon, cultural and language differences, and the need for effective communication of material properties. We have discussed various strategies to overcome these challenges, such as utilizing visual aids, fostering continuous education, and leveraging collaborative decision-making tools. Additionally, the invaluable role of material experts in guiding the selection process cannot be overstated, as their specialized insights ensure the alignment of material properties with project requirements.
In essence, successful material selection is a collaborative effort that blends technical expertise with clear, accessible communication. By adopting best practices and fostering an environment of continuous learning and collaboration, teams can make more informed decisions, mitigate risks, and drive innovation in product design. The journey from understanding material specifications to their practical application is complex, but with the right approach, it becomes a pathway to creating superior, reliable, and cost-effective products.
コメント